Quantum Supremacy A New Era of Computing
Quantum supremacy marks a pivotal moment in computing history, promising to revolutionize fields ranging from medicine to materials science. It signifies the point where a quantum computer can solve a problem that is practically impossible for even the most powerful classical computers. This leap forward isn’t just about faster calculations; it’s about accessing entirely new computational landscapes, unlocking solutions to problems previously considered intractable.
Understanding quantum supremacy requires delving into the fundamental differences between quantum and classical computation, exploring the technological marvels that make it possible, and acknowledging the ongoing debates and challenges surrounding its development.
The journey towards quantum supremacy has been paved with significant milestones, from the theoretical groundwork laid by pioneers in quantum mechanics to the construction of increasingly sophisticated quantum computers. This progress hasn’t been without its hurdles; building and maintaining stable quantum systems presents enormous technical challenges. However, the potential rewards – the ability to tackle previously unsolvable problems – are driving relentless innovation in this exciting field.
Defining Quantum Supremacy
Quantum supremacy is a milestone in the field of quantum computing. It signifies the point at which a quantum computer performs a calculation that is demonstrably beyond the capabilities of even the most powerful classical computers. In simpler terms, it’s when a quantum computer can solve a problem that a regular computer just can’t handle, not because of a lack of processing power, but because of a fundamental difference in how they operate.Quantum supremacy isn’t about solving everyday problems faster; it’s about proving the potential of quantum computers to tackle specific, complex tasks that are currently intractable for classical computers.
Think of it as a proof-of-concept, a demonstration that quantum computing is a viable path towards solving problems previously considered unsolvable.
Historical Milestones in Demonstrating Quantum Supremacy
The quest for quantum supremacy has been a journey marked by incremental advancements. Google’s 2019 claim of achieving quantum supremacy with their Sycamore processor, performing a calculation in 200 seconds that would take the world’s most powerful supercomputers thousands of years, is a significant milestone. This involved a specific, albeit artificial, computational task designed to highlight the quantum computer’s advantage.
Other groups, including those at IBM and various academic institutions, have continued to push the boundaries, focusing on developing more robust and error-corrected quantum computers, improving the algorithms used, and striving for more practical demonstrations of quantum advantage in real-world applications. The field is rapidly evolving, with constant progress being made in both hardware and software.
Quantum Supremacy Versus Classical Computation
The core difference lies in how they process information. Classical computers use bits, which represent either a 0 or a 1. Quantum computers use qubits, which can represent 0, 1, or a superposition of both simultaneously. This superposition, combined with the principles of quantum entanglement, allows quantum computers to explore many possibilities at once, providing a massive speed advantage for certain types of problems.
While classical computers excel at many tasks, they struggle with problems involving large numbers of variables and complex interactions, such as simulating molecular behavior or breaking certain types of encryption. Quantum computers offer a potential pathway to solve these problems efficiently.
Key Differences Between Quantum and Classical Algorithms
Classical algorithms are sequential and deterministic; they follow a set of instructions step-by-step, producing a predictable output. Quantum algorithms, however, leverage quantum phenomena like superposition and entanglement to perform computations in a fundamentally different way. For instance, Shor’s algorithm, a quantum algorithm, can factor large numbers exponentially faster than the best known classical algorithms, posing a threat to widely used encryption methods.
Grover’s algorithm, another notable quantum algorithm, offers a quadratic speedup for searching unsorted databases compared to classical search algorithms. These differences stem from the inherent capabilities of qubits to exist in multiple states simultaneously and to be entangled, allowing for parallel processing and exploring a vast solution space concurrently.
Technological Requirements for Quantum Supremacy
Achieving quantum supremacy demands significant advancements in several key areas of quantum computing technology. The creation of a quantum computer capable of outperforming even the most powerful classical supercomputers requires not only innovative designs but also meticulous control and manipulation of incredibly fragile quantum systems. This section delves into the specific hardware, challenges, and qubit types crucial for reaching this milestone.
Essential Hardware Components
Building a quantum computer capable of demonstrating quantum supremacy necessitates a complex interplay of various highly specialized hardware components. These components work in concert to create, control, and measure the quantum states of qubits, the fundamental building blocks of quantum computation. Key elements include highly precise control electronics for manipulating individual qubits, cryogenic systems to maintain extremely low temperatures (typically near absolute zero), high-fidelity quantum gates for implementing quantum algorithms, and efficient measurement systems to extract the results of computations.
Furthermore, sophisticated error correction mechanisms are crucial to mitigate the effects of noise and decoherence, which are significant hurdles in maintaining stable quantum states. The integration of all these components into a functioning, scalable system presents a formidable engineering challenge.
Challenges in Building and Maintaining Stable Quantum Systems
One of the most significant obstacles in building quantum computers is the inherent fragility of quantum states. Qubits are susceptible to decoherence, where their quantum properties are lost due to interactions with the environment. This interaction can be caused by thermal fluctuations, electromagnetic noise, or other sources of interference. Maintaining the coherence of qubits for sufficiently long periods is crucial for performing complex computations.
Quantum supremacy, the point where quantum computers outperform classical ones, is a huge goal. Achieving this relies heavily on advancements in Quantum AI hardware development and its challenges in scalability, as discussed in this insightful article: Quantum AI hardware development and its challenges in scalability. Overcoming these scalability hurdles is crucial for truly realizing the potential of quantum supremacy and its transformative applications.
Another challenge is scalability: building a quantum computer with a sufficient number of qubits to tackle complex problems is incredibly difficult. The complexity and cost of manufacturing and controlling a large number of qubits increase exponentially. Finally, error correction is vital because even minor errors can accumulate and lead to incorrect results. Developing efficient and robust error correction codes and implementing them in hardware are active areas of research.
Google’s Sycamore processor, for example, while demonstrating quantum supremacy, suffered from a high error rate.
Types of Qubits and Their Properties
Several different types of qubits are being pursued, each with its own advantages and disadvantages. Superconducting qubits, like those used in Google’s Sycamore processor, are fabricated using superconducting circuits and are relatively mature. They offer good coherence times and can be controlled with high precision. However, they require cryogenic cooling to extremely low temperatures. Trapped ion qubits utilize individual ions trapped in electromagnetic fields.
These qubits boast long coherence times and high fidelity gates, but scaling them up to a large number of qubits is challenging. Photonic qubits, based on photons, offer the potential for long-distance communication and entanglement, but controlling and manipulating them is more complex. Neutral atom qubits, using individually trapped neutral atoms, provide another promising approach with good scalability potential and long coherence times.
The choice of qubit type often depends on the specific application and the trade-offs between coherence time, scalability, and controllability.
Hypothetical Architecture for a Quantum Supremacy Computer
A hypothetical architecture for a quantum computer capable of demonstrating quantum supremacy might incorporate a modular design. Imagine a system built from interconnected chips, each containing a relatively small number (e.g., 100-500) of superconducting qubits. These chips would be connected via high-bandwidth communication links allowing for the entanglement and manipulation of qubits across multiple chips. Each chip would have its own dedicated control electronics and cryogenic cooling system.
Sophisticated error correction codes would be implemented using a combination of hardware and software techniques. The overall system would be controlled by a powerful classical computer responsible for managing the quantum computation, compiling quantum algorithms, and processing the measurement results. This modular approach would facilitate scalability and allow for the gradual increase in the number of qubits as technology improves.
The architecture would prioritize minimizing noise and decoherence, maximizing qubit coherence times, and ensuring high-fidelity quantum gates. This hypothetical architecture reflects current trends and technological capabilities in the field, drawing inspiration from existing quantum computing platforms while incorporating potential future advancements.
Demonstrations and Experiments
Claims of quantum supremacy represent a significant milestone in the development of quantum computing. These claims, however, are not without controversy, stemming from both the complexity of the experiments and the evolving understanding of quantum computation itself. The demonstrations involve highly specialized hardware and software, pushing the boundaries of what’s currently technologically feasible.Demonstrations of quantum supremacy aim to show that a quantum computer can perform a specific calculation that is practically impossible for even the most powerful classical computers.
This isn’t about solving every problem faster, but rather demonstrating a clear advantage in a specific, albeit artificial, computational task. The choice of these tasks is crucial, and the criteria for success are constantly debated within the scientific community.
Examples of Quantum Supremacy Experiments
The following table summarizes some key experiments that have claimed to demonstrate quantum supremacy, chronologically ordered to show the progression of capabilities:
Experiment Name | Research Team | Year | Key Findings |
---|---|---|---|
Quantum Supremacy Using a Programmable Superconducting Processor | Google AI Quantum | 2019 | Google’s Sycamore processor performed a calculation in 200 seconds that they estimated would take the most powerful classical supercomputers 10,000 years. This calculation involved sampling the output of a pseudo-random quantum circuit. The claim was met with immediate scrutiny and debate. |
Experimental Quantum Speedup in Random Circuit Sampling Using a 64-Superconducting-Qubit Processor | University of Science and Technology of China | 2020 | A team in China used a 64-qubit superconducting processor called “Jiuzhang” to achieve a quantum computational advantage in a similar random sampling problem. Their claim focused on a faster computational speed compared to classical approaches. The specific task was also different from Google’s experiment. |
Achieving Quantum Supremacy with 127-Qubit Sycamore Processor | Google AI Quantum | 2023 | Google announced a new experiment using a more advanced 127-qubit Sycamore processor, significantly extending their earlier work. This experiment further demonstrated quantum computational advantage in a more complex random sampling task. The improvement highlights the progress in both qubit number and control. |
(Future Experiment Placeholder) | (To be determined) | (Future Year) | Further advancements in quantum computing technology will undoubtedly lead to new experiments aiming to demonstrate even more robust forms of quantum supremacy. These experiments might involve different computational tasks or address criticisms of previous claims. |
Comparison of Computational Tasks
The computational tasks used in these experiments, primarily variations of random circuit sampling, were chosen for their inherent difficulty for classical computers to simulate efficiently. The randomness is key, making it difficult to find shortcuts or approximations. However, the choice of these specific tasks has been a source of criticism, with some arguing that they lack practical relevance beyond demonstrating the potential of quantum computers.
Future demonstrations may involve more practically relevant problems, though these are more challenging to implement and verify.
Criticisms and Controversies
The claims of quantum supremacy have sparked considerable debate. Critics have questioned the validity of the claims, focusing on several points:* Classical simulation limitations: The difficulty of simulating quantum circuits classically is still a matter of ongoing research. Improvements in classical algorithms and hardware could potentially reduce the claimed computational advantage.
Task relevance
Quantum supremacy, the point where quantum computers outperform classical ones, is a huge leap forward. However, this power raises serious questions, especially when considering its application in AI. The potential for incredibly advanced autonomous weapons systems is a key concern, as discussed in this article: The ethical implications of using quantum AI in autonomous weapons systems. Therefore, responsible development of quantum supremacy is crucial to avoid catastrophic misuse.
The chosen tasks, while demonstrating quantum computational advantage, often lack direct practical applications.
Verification challenges
Verifying the results of these experiments requires sophisticated methods and independent confirmation. The complexity of the experiments makes it challenging to rule out all sources of error.
Definition of supremacy
The very definition of quantum supremacy is subject to ongoing discussion. Different researchers may have varying criteria for what constitutes a genuine demonstration of quantum advantage.These criticisms highlight the need for continued research and development in both quantum and classical computing to establish a clearer and more universally accepted definition of quantum supremacy and its implications.
Applications and Implications
Quantum supremacy, the ability of a quantum computer to solve a problem that is intractable for even the most powerful classical computers, opens up a vast landscape of potential applications and profound implications across various fields. The ability to harness the power of quantum mechanics for computation promises to revolutionize our understanding of the world and our ability to solve complex problems currently beyond our reach.The impact of quantum supremacy extends far beyond theoretical advancements; it holds the potential to reshape industries and redefine technological capabilities.
While still in its early stages, the implications are already being felt, driving significant investment and research into this transformative technology.
Potential Applications Leveraging Quantum Supremacy
Quantum computers, once they reach a level of maturity beyond demonstration, are expected to excel in several areas. Their ability to explore a vast number of possibilities simultaneously makes them ideal for tackling problems that require exponential computational resources for classical computers. For example, drug discovery and materials science stand to benefit greatly from the ability to simulate molecular interactions with far greater accuracy and speed than is currently possible.
Quantum supremacy, the point where quantum computers outperform classical ones, is a huge step forward. This breakthrough opens doors to incredibly complex calculations, as explored in detail by this article on The role of quantum AI in personalized medicine and healthcare , highlighting its potential in drug discovery. Ultimately, achieving quantum supremacy will accelerate advancements across numerous fields, including personalized medicine.
Furthermore, quantum algorithms like Shor’s algorithm could revolutionize cryptography by breaking widely used encryption methods, necessitating the development of quantum-resistant cryptography. Finally, optimization problems, crucial in logistics, finance, and artificial intelligence, could be solved more efficiently.
Impact on Medicine, Materials Science, and Cryptography
The pharmaceutical industry could experience a paradigm shift. Quantum computers could simulate the behavior of molecules with unprecedented accuracy, accelerating drug discovery and development. This would allow researchers to design more effective drugs with fewer side effects and significantly reduce the time and cost associated with bringing new medications to market. Imagine personalized medicine, where drugs are tailored to an individual’s genetic makeup, becoming a reality.Materials science is another field poised for transformation.
Quantum simulations could enable the design of novel materials with specific properties, leading to advancements in areas such as energy storage, electronics, and construction. For example, the development of more efficient solar cells or superconductors with higher critical temperatures could revolutionize energy production and transmission.Cryptography, as mentioned earlier, faces a significant challenge. Shor’s algorithm, a quantum algorithm, poses a serious threat to current public-key cryptography systems.
This necessitates a global effort to develop and implement quantum-resistant cryptographic techniques to secure data in the quantum computing era. The development and implementation of these new cryptographic methods is crucial to maintaining data security in the face of potential threats.
Quantum supremacy, the point where quantum computers outperform classical ones, is a significant milestone. This achievement opens doors to tackling previously unsolvable problems, and a prime example lies in logistics. Check out this article on Quantum AI’s potential in solving optimization problems in logistics to see how this works. Ultimately, the practical applications of quantum supremacy, especially in complex optimization, are incredibly promising.
Currently Intractable Problems Potentially Solvable with Quantum Computers
Several problems that are currently computationally infeasible for classical computers may become solvable with sufficiently advanced quantum computers. These include:* Protein folding: Predicting the three-dimensional structure of proteins is crucial for understanding biological processes and developing new drugs. Classical computers struggle with the complexity of this problem, while quantum computers offer a potential solution.
Optimization problems
Many real-world problems, such as traffic flow optimization, financial portfolio optimization, and supply chain management, can be formulated as optimization problems. Quantum algorithms offer the potential to find better solutions faster than classical algorithms.
Materials simulation
Accurately simulating the behavior of materials at the atomic level is essential for designing new materials with desired properties. Quantum computers can provide more accurate and efficient simulations than classical computers.
Database searching
Searching large databases for specific information can be time-consuming for classical computers. Quantum algorithms offer the potential to significantly speed up this process.
Long-Term Societal Implications of Widespread Quantum Computing
The widespread adoption of quantum computing will have profound and far-reaching societal implications. These include:
- Economic disruption: Quantum computing could lead to significant job displacement in some sectors, while creating new opportunities in others. Retraining and adaptation will be crucial to manage this transition.
- National security implications: The potential for breaking current encryption methods raises concerns about national security and data privacy. The development and deployment of quantum-resistant cryptography is paramount.
- Ethical considerations: The power of quantum computing raises ethical questions about its use in areas such as surveillance, genetic engineering, and autonomous weapons systems. Careful consideration and responsible development are crucial.
- Scientific breakthroughs: Quantum computing has the potential to accelerate scientific discovery across various fields, leading to breakthroughs in medicine, materials science, and other areas. This could significantly improve human lives.
- Increased computational power: The exponential increase in computational power will open up new possibilities for solving complex problems, leading to advancements in various fields.
Future Directions and Challenges
The journey towards practical quantum computation is far from over. While demonstrations of quantum supremacy have been achieved, significant technological hurdles remain before we can harness the full potential of quantum computers for real-world applications. Overcoming these challenges requires a multi-pronged approach encompassing hardware improvements, error correction advancements, and the development of new quantum algorithms.Technological hurdles currently impede the development of large-scale, fault-tolerant quantum computers.
These limitations primarily stem from the fragility of quantum states, the difficulty in maintaining coherence, and the challenges in scaling up the number of qubits while preserving their quality. The current generation of quantum computers, even the most advanced, are prone to errors and limited in their computational power compared to what is theoretically possible.
Remaining Technological Hurdles
The path to practical quantum computation is paved with significant technological challenges. Improving qubit coherence times is paramount; longer coherence times allow for more complex computations before errors accumulate. Currently, the decoherence rates of qubits limit the length of calculations that can be performed reliably. Furthermore, achieving high-fidelity quantum gates is crucial. These gates manipulate the qubits to perform computations, and any inaccuracies in their operation propagate errors throughout the calculation.
Finally, scaling up the number of qubits while maintaining their quality and control is a major engineering challenge. Current quantum computers have only a limited number of qubits, far fewer than what would be required for many practical applications.
Research Areas Requiring Further Development
Several key research areas need significant advancements to improve quantum computer performance. Developing new qubit technologies is vital; exploring different physical implementations, such as trapped ions, superconducting circuits, and photonic qubits, is crucial to find the most robust and scalable approach. Simultaneously, improving quantum error correction codes is essential. These codes protect quantum information from noise and errors, allowing for fault-tolerant quantum computation.
The development of more efficient and robust error correction techniques is critical for scaling up quantum computers. Finally, designing new quantum algorithms tailored to the strengths of quantum computers is essential. While some algorithms, like Shor’s algorithm for factoring large numbers, have been known for some time, the development of new algorithms for specific applications remains an active area of research.
Roadmap for Achieving Fault-Tolerant Quantum Computation
A roadmap for achieving fault-tolerant quantum computation involves several key steps. First, the development and improvement of high-fidelity qubits with long coherence times is crucial. This requires significant advances in materials science, nanofabrication, and control techniques. Second, the development and implementation of efficient quantum error correction codes is paramount. This involves theoretical research on new codes and experimental validation of their effectiveness.
Third, the development of scalable architectures for building large-scale quantum computers is necessary. This includes designing efficient ways to connect and control many qubits. Finally, the development of robust software and programming tools is crucial to make quantum computers accessible and usable. This includes the development of quantum programming languages and compilers. The timeline for achieving fault-tolerance is uncertain, but progress in these areas is crucial.
Predictions for the Timeline of Widespread Quantum Computational Supremacy
Predicting the timeline for widespread quantum computational supremacy is challenging, akin to predicting the exact date the internet would become ubiquitous. While some experts suggest that fault-tolerant quantum computers may be decades away, others believe that significant advancements in specific applications could emerge sooner. For example, quantum annealing machines, a specialized type of quantum computer, are already being used for optimization problems in areas such as materials science and logistics.
However, achieving general-purpose fault-tolerant quantum computation that surpasses classical computers for a wide range of tasks is likely a longer-term goal. Considering the rapid pace of technological advancements in the field, it’s reasonable to anticipate significant progress within the next 10-20 years, with potentially widespread adoption following within a subsequent decade or two, depending on the rate of technological breakthroughs and the successful resolution of the challenges Artikeld above.
The development of error correction schemes, for example, directly impacts this timeline. More efficient schemes will accelerate the process, while less effective ones could delay it significantly. The analogy to the internet is relevant because initial development was slow, but once certain critical thresholds were crossed, adoption accelerated dramatically.
Illustrative Example: Quantum Supremacy in Factoring
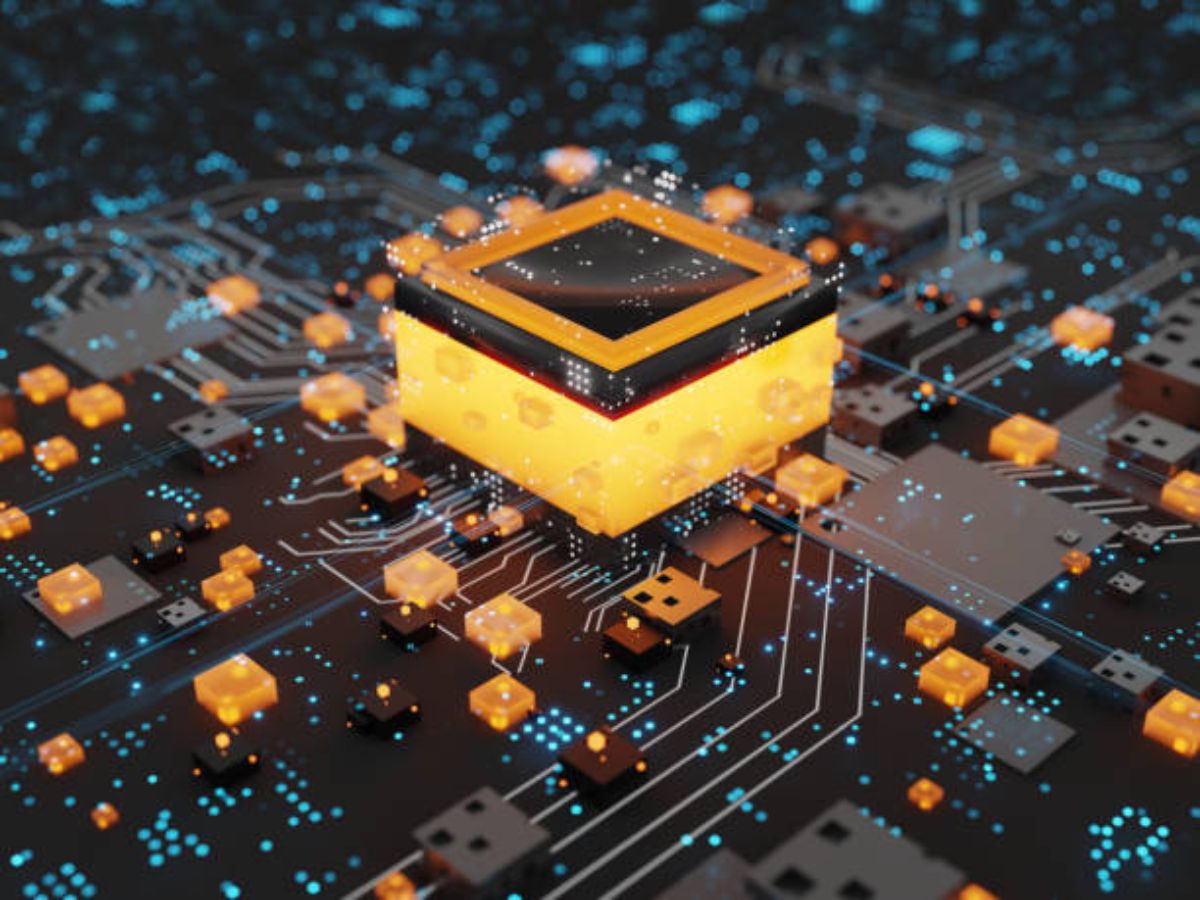
Source: abplive.com
Shor’s algorithm stands as a compelling example of quantum supremacy, showcasing the potential of quantum computers to solve problems intractable for even the most powerful classical computers. This algorithm focuses on the notoriously difficult problem of integer factorization – breaking a large number down into its prime factors. Its efficiency stems from the unique properties of quantum mechanics, offering a significant speedup compared to classical approaches.Shor’s Algorithm and its Potential to Factor Large NumbersShor’s algorithm leverages quantum Fourier transforms and modular arithmetic to efficiently find the prime factors of a large composite number.
It’s a probabilistic algorithm, meaning it doesn’t guarantee a solution on every run, but the probability of success increases with repeated executions. The core of the algorithm involves finding the period of a function related to the number being factored. This period is then used to determine the factors. The quantum computer’s ability to exist in a superposition of states and perform quantum computations allows it to explore many possible periods simultaneously, leading to an exponential speedup over classical algorithms.
Quantum Advantage Demonstrated by Shor’s Algorithm
The quantum advantage offered by Shor’s algorithm is dramatic. Classical algorithms, like the General Number Field Sieve (GNFS), exhibit exponential runtime complexity when factoring large numbers. This means the time required to factor a number increases exponentially with the number of digits. In contrast, Shor’s algorithm runs in polynomial time, meaning the increase in computation time is far less dramatic as the number of digits grows.
This difference in scaling is what makes Shor’s algorithm so significant. It’s not just a small improvement; it’s a fundamental shift in computational capability.
Comparison of Shor’s Algorithm and Classical Algorithms
Let’s consider factoring a 2048-bit number (approximately 617 decimal digits), a common key size in RSA cryptography. The best known classical algorithms would require an impractical amount of time to factor this number – potentially thousands of years even with the most powerful supercomputers available today. Shor’s algorithm, implemented on a sufficiently large and fault-tolerant quantum computer, could potentially factor this number in a matter of hours or days.
The computational advantage of Shor’s algorithm over classical algorithms for factoring large numbers is exponential. While classical algorithms scale exponentially with the size of the number, Shor’s algorithm scales polynomially, leading to a dramatic reduction in computation time for large inputs.
Impact of Shor’s Algorithm Speedup on Cryptography
The speedup offered by Shor’s algorithm poses a significant threat to widely used public-key cryptosystems, such as RSA, which rely on the difficulty of factoring large numbers. If large-scale quantum computers become a reality, these cryptosystems would become vulnerable, as Shor’s algorithm could break them relatively quickly. This necessitates the development of post-quantum cryptography – cryptographic algorithms that are secure against attacks from both classical and quantum computers.
Research in this area is actively underway to ensure the continued security of digital communication in the age of quantum computing.
Wrap-Up
The pursuit of quantum supremacy is a testament to human ingenuity and our relentless quest to push the boundaries of computation. While significant challenges remain, the progress made to date is undeniably impressive. The potential applications of quantum computers are vast, promising breakthroughs in various fields. However, it’s crucial to approach this transformative technology responsibly, carefully considering its ethical and societal implications.
The future of quantum computing is bright, but its realization will require continued collaboration, innovation, and a commitment to responsible development.
Key Questions Answered
What is a qubit?
A qubit is the fundamental unit of quantum information, analogous to a bit in classical computing. Unlike bits, which can be either 0 or 1, qubits can exist in a superposition, representing both 0 and 1 simultaneously.
What are the limitations of current quantum computers?
Current quantum computers are prone to errors due to decoherence (loss of quantum information) and are limited in the number of qubits they can reliably control. They also lack the fault tolerance necessary for large-scale computations.
When will quantum computers be widely available?
Predicting the timeline for widespread availability is difficult. While significant progress is being made, widespread availability of powerful, fault-tolerant quantum computers is likely still many years away.
How does quantum supremacy impact cybersecurity?
Quantum computers, particularly with Shor’s algorithm, pose a significant threat to current encryption methods. New, quantum-resistant cryptographic techniques are being developed to address this challenge.
What is the difference between quantum advantage and quantum supremacy?
Quantum supremacy refers to demonstrating that a quantum computer can solve a specific problem faster than any classical computer. Quantum advantage is a broader term referring to any situation where a quantum computer offers a practical advantage over classical computers, even if not definitively faster for all problems.